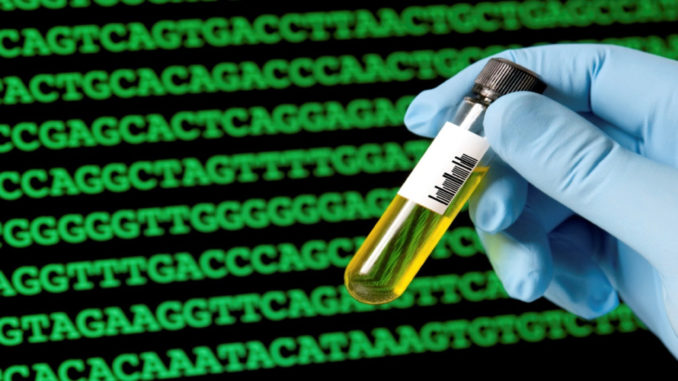
The quest to expand the genetic alphabet has been a fascinating journey, delving deep into the very essence of life’s building blocks. At its core, this field of research aims to move beyond the four standard DNA bases – adenine (A), thymine (T), guanine (G), and cytosine (C) – and introduce new, synthetic bases that can be incorporated into the genetic code.
The science behind this endeavor is complex and multifaceted. To create synthetic bases, researchers must first design molecules that can mimic the structure and function of natural bases. These synthetic bases must be able to form stable base pairs with each other, much like A pairs with T and G pairs with C in natural DNA. However, the synthetic bases must also be distinct enough to avoid cross-pairing with the natural bases, ensuring the integrity of the expanded genetic system.
One of the early breakthroughs in this field came from Floyd Romesberg’s team at the Scripps Research Institute. In 2014, they successfully incorporated two synthetic bases, d5SICS and dNaM, into the DNA of E. coli bacteria. These bases, known as “unnatural base pairs” (UBPs), were designed to pair with each other but not with the natural bases. To achieve this, the researchers used a combination of hydrophobic and hydrogen-bonding interactions to create a stable pairing between d5SICS and dNaM.
The incorporation of these synthetic bases into living organisms opened up new possibilities for protein engineering and the production of novel compounds. By expanding the genetic alphabet, researchers could potentially code for a wider range of amino acids, leading to the creation of proteins with enhanced or entirely new functions.
As the field progressed, other synthetic base pairs were developed, each with its own unique properties. Steven Benner, Elisa Biondi, and their colleagues at the Foundation for Applied Molecular Evolution introduced the “Hachimoji DNA” system in 2019, which included eight bases: the four natural ones and four synthetic bases (P, B, Z, and S). The synthetic bases in Hachimoji DNA were designed to form stable base pairs (P with Z and B with S) while avoiding cross-pairing with the natural bases. This expanded genetic alphabet demonstrated the potential for creating stable double helices and opened up new avenues for applications in drug discovery and biosensing.
The science behind these synthetic bases relies heavily on the principles of molecular recognition and the precise control of hydrogen bonding, hydrophobic interactions, and shape complementarity. By carefully designing the structure of the synthetic bases, researchers can create molecules that mimic the function of natural bases while also introducing new properties and capabilities.
In addition to the development of synthetic bases, researchers have also been working on creating the necessary molecular machinery to replicate, transcribe, and translate expanded genetic alphabets. This includes the development of engineered polymerases that can efficiently incorporate synthetic bases into DNA and RNA strands, as well as the creation of ribosomes that can translate expanded codons into novel amino acids.
The potential applications of expanded genetic alphabets are vast and far-reaching. In medicine, synthetic bases could enable the development of new drugs and therapies that target specific genetic sequences or protein structures. In biotechnology, expanded genetic systems could lead to the creation of novel enzymes and biosensors with enhanced capabilities. And in materials science, synthetic DNA could be used to create programmable nanostructures and advanced materials with precise control over their properties.
However, the science behind expanding the genetic alphabet is still in its early stages, and there are many challenges that must be overcome. These include improving the stability and replication fidelity of synthetic base pairs, developing robust methods for the large-scale synthesis of synthetic DNA, and understanding the potential impacts of expanded genetic systems on natural ecosystems.
Despite these challenges, the scientific community remains excited about the potential of expanded genetic alphabets. By pushing the boundaries of what is possible with the building blocks of life, researchers are not only advancing our understanding of the fundamental principles of biology but also opening up new frontiers in medicine, biotechnology, and materials science.
As this field continues to evolve, it is essential for researchers to collaborate and build upon each other’s work. The contributions of pioneers like Floyd Romesberg, Steven Benner, Elisa Biondi, Philipp Holliger, Ichiro Hirao, and Eric Kool have laid the foundation for this exciting area of research, and their ongoing efforts continue to drive the field forward.
To stay at the forefront of this rapidly advancing field, it is crucial to engage with the scientific community and stay informed about the latest developments. Following leading researchers on Twitter, such as @FloydRomesberg, StevenBenner (no known Twitter account), @philholliger, @ihirao, and @koolchemistry, is an excellent way to stay connected and up-to-date with the newest breakthroughs and discoveries in the world of synthetic biology and expanded genetic alphabets.
As we unravel the science behind synthetic bases and expanded genetic systems, we are not only expanding our understanding of life’s fundamental building blocks but also unlocking new possibilities for the future of medicine, biotechnology, and beyond. The journey to expand the genetic code is an ongoing one, full of challenges and opportunities, and the scientific community stands poised to explore this exciting new frontier.